The first underlying premise of structural engineering is a good, solid foundation. Level and plumb, with enough capacity to support the weight and loads of the structure it supports. If there is trouble with the foundation, there will be trouble with the structure. The second premise is Newton’s Second Law of Motion, which states that the sum of the forces acting on an object is equal to the change in the object’s momentum with time. We structural engineers just simplify this to say that the sum of the forces acting on an object is equal to zero, since structures have no momentum since they don’t move.
From antiquity, engineers have assumed that the ground we build on will remain solid forever and will never move. And those two assumptions have, in general, been good ones to make. Look at the castles across Europe. Look at the pyramids in Egypt. Thousands of years old and they are still standing. But what if I were to tell you a secret? What if I were to tell you that the ground we build on is not solid and is constantly moving?
Of course, the amount of movement depends on a lot of variables. What type of soil is present? Where in the world are we located? Our knowledge of the Earth’s constant movement has come a long way in a short amount of time, as is our ability to predict and design for that movement. Engineers call this seismic engineering.
An earthquake is the abrupt movement of the ground. It is an event. Most of us have experienced this event, those who haven’t have seen its effects on the news. What we consider solid ground is actually a series of thick islands floating on a sea of red hot magma. And these islands are being tossed about on this sea and they are forever bashing and colliding into one another. When they collide, violent things happen. Imagine a car accident on a much larger scale.
When these islands collide, everything that sits on them gets tossed about. Seventy percent of the Earth’s surface is covered by water. And you know what happens when someone bumps into you while you’re holding that boiling hot cup of coffee in the morning. The sloshing caused by an earthquake can send huge waves toward land. If the earthquake is large enough, it can cause landslides, cracks in the ground and it can even lay waste to entire cities.
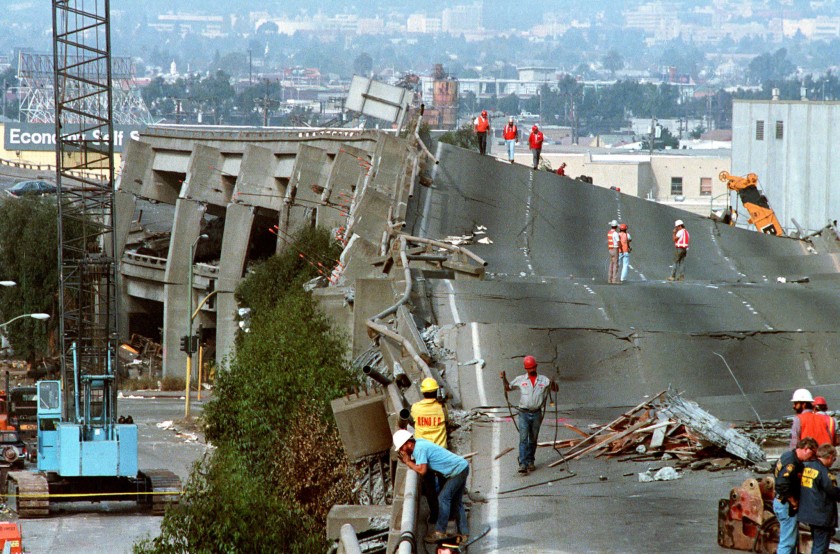
But for years, our understanding of this phenomena was feeble at best. A series of disastrous earthquakes in California made the engineering world stand up and pay attention. Scientists began to study the problem from various angles. Why was this occurring in certain areas and not others? Why do some structures collapse? How can we predict the load demands from an earthquake?
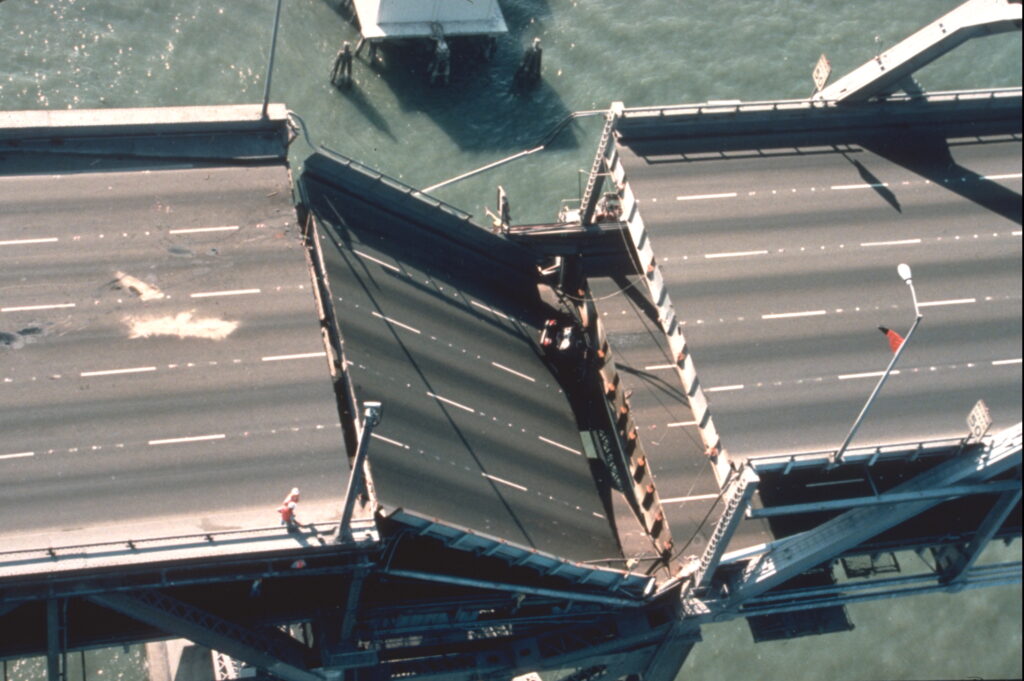
Starting the late 1970’s, seismic design – that is the way engineers attack the problem of designing for these events – took a sudden change. Before this, our concerns were simply vertical loads, those caused by gravity. The only lateral load we considered was wind. But once we began to understand how structures behave in an earthquake, our approach began to change. Detailing of structural elements and connections changed. More thought was devoted to the flow of forces laterally through a structure. The principles of structural analysis were all there, just applied in a new way.
Like the year one, the change over from B.C. reckoning to A.D. reckoning, most structures built from the late 1970’s and on are generally considered to be “designed for seismic.” While the loading and our fundamental understanding may have changed between then and now, the detailing in the structure is generally considered far superior in these structures compared to those of the pre-seismic design era.
In the world of bridge engineering, engineers are now trained early on in seismic design. Single mode two-dimensional analysis models are common fare on engineering tests. In practice, multi-mode response spectrum analysis is the tool of choice for demand models. Over the years, capacity models have advanced from force based to displacement based as computers have advanced. Our ability to study structure behavior to the minutiae has revolutionized our practice.
Now that’s all well and good for new bridges. But what about those bridges still around from the pre-seismic design era? What do we do about them?
Not to fear! Engineers have given a name to the conundrum. Seismic retrofit. As detailed in my last article, The Need for Infrastructure Spending, the average age of the bridges in Washington State is 48 years. Think about it, of the 3000 bridges in Washington, 1500 of them were built prior to 1972 – definitely pre-seismic design. That means 1500 bridges with poor seismic detailing, with little thought given to a lateral force load path. These bridges need a seismic retrofit.
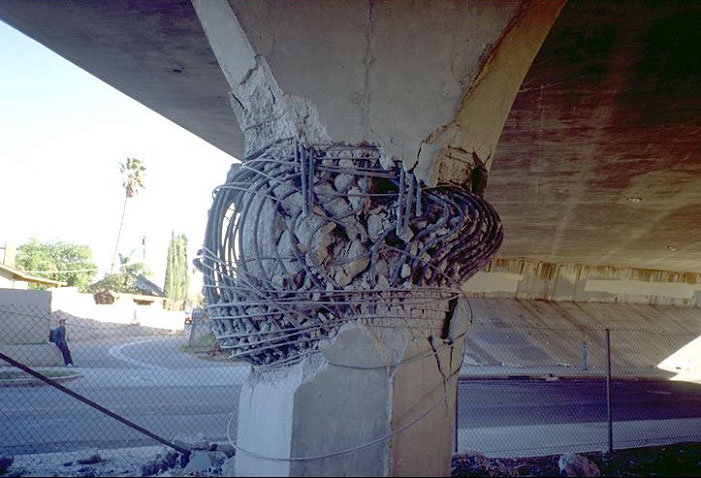
A seismic retrofit program has been underway in the Western States for years. This program has sought to enhance the safety of pre-seismic design structures in a variety of ways. Some bridges are retrofitted by strengthening the lateral force resisting system to withstand some level of seismic loading. Other bridges are retrofitted by adding some means of lateral restraint to prevent collapse in an earthquake. Still others seek to modify the way the bridge behaves by changing certain characteristics of the bridge. While the method may vary, the outcome is the same – no collapse.
Like the infrastructure problems that we face, similar problems effect the retrofit of seismically vulnerable bridges. While this problem is more regionally affected rather than nationally, the problem of funding still exists. This is especially true when there are competing programs all angling for the same limited funds. The key to overcoming this is prioritization of the needs.
Many states have identified life line routes in the event of emergencies. The actual definition of what a life line is and how it may be utilized vary by jurisdiction, but in general they are considered the main transportation arteries for incoming aid or for mass evacuation. In short, these are essential routes that must be open after an emergency.
Bridge design codes give three different design levels – normal, essential and critical. For each level, a different set of criteria can be set for the design of structures in those categories. Again, the definition of a bridge at one of these levels is left to the jurisdiction. In Washington, the critical designation is reserved for high value assets whose replacement cost and down time impact on the traveling public would be immense. Such structures are designed to a higher level of seismic design, often a multi-tier design effort with specific strain limits on steel, specific crack widths for concrete and limited amounts of ductility. The goal here is to have the structure serviceable – open for traffic – shortly after an event occurs with little to no repairs required.
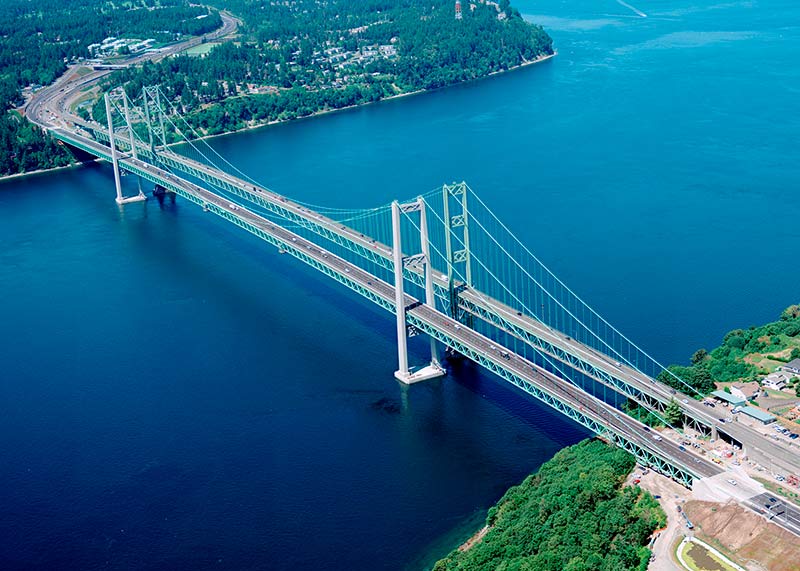
Essential bridges are typically those located on an identified lifeline route. These bridges will be designed similar to a critical bridge, but with less stringent ductility considerations. The goal here is to have these structures remain serviceable at the lower level design earthquake yet not collapse at the upper level.
Normal bridges are those that are neither considered critical or essential. Really just a regular bridge not on a life line route. These bridges are typically design to a single tier design event with the goal being no collapse.
In a perfect world, all bridges would be critical and all would remain in service after an earthquake. However, this just isn’t practical. It is acknowledged by many decision makers that if a large subduction zone earthquake were to hit the Seattle area as has been predicted, the entire region would be on its knees. Even if all of the bridges were to survive such an event, the approaching road fills may have liquefied, buildings will have collapsed, power lines will be down, gas lines will have ruptured. Even if the bridge was still standing, it is unlikely that you would be able to reach it.
And how much do we really know about that next earthquake? When will it strike? Where will it strike? How bad will it be? In real terms, we have no idea. Engineers take a probabilistic approach to seismic design. They look at return periods – what is the likelihood of an earthquake of that size happening again in a certain timeframe. For normal bridges in Washington, this is an earthquake that has a 7% chance of being exceeded in 75 years, which is the design life of a bridge under current codes. If you do the math that is an earthquake that statistically happens every 1000 years.
Some jurisdictions design for a 2500 year event. Some design for a 500 year event. But these are all guesses. It is a gamble that the largest earthquake that will occur in that bridge’s life will be the one that the engineer designed it for. Designing for a lower level earthquake may leave you open to some amount of risk. Designing for a higher level earthquake could bust your budget. But one thing is clear – a bridge designed for some level of earthquake is better than a bridge not designed for one at all.
Great article & love the style!
An excellent share, I just provided this onto a colleague that was doing a little analysis on this. And also he actually purchased me morning meal since I discovered it for him. smile. So let me rephrase that: Thnx for the treat! Yet yeah Thnkx for spending the time to discuss this, I feel strongly regarding it as well as love finding out more on this topic. Preferably, as you come to be competence, would certainly you mind upgrading your blog with even more information? It is highly helpful for me. Big thumb up for this article!
Your place is valueble for me. Thanks !?
Hello! I just wish to provide a big thumbs up for the terrific information you have here on this message. I will be returning to your blog for even more quickly.
really good blog post, i certainly love this site, keep it