A bridge can be divided into two parts, the superstructure and the substructure. If we look at the make-up of these two words, we see they describe a bridge as a structure in a very literal manner. A superstructure is the joining of ‘super,’ meaning that which is above and structure. So the superstructure is the upper portion of the bridge structure. A substructure joins the prefix ‘sub,’ meaning that which is below and structure. So the substructure is the lower portion of the structure. Its bridge anatomy!
The division between the superstructure and the substructure is a key dividing line for bridge design. Often on large bridge projects, one group will design the former while another will design the latter. On smaller bridges, one designer may design both. In some bridges, the distinction between super and sub is an academic one – for instance, in floating bridges.
And in between the two is another important component to any bridge – the bearings. Bearings are devices which serve two main functions in a bridge, they constrain motion at a joint and they relieve friction between two surfaces. Bearings in this sense can be extremely simple. In many high seismic areas, the joint between the superstructure and the substructure may be constrained in all six degrees of freedom such as in an integral design. The two are fused together and act as one.
More sophisticated and complex bearings are utilized where necessary. In general, there are two main design features we must consider in the design of a bridge bearing. First, how much axial load can the bearing support. This will often be a function of the bridge type and layout as much as the type of bearing used. Second, how much motion is required of the bearing. More flexible bridges require greater ranges of motion which will determine the bearing type.
The first bearing to be considered is the elastomeric bearing. Older versions were unreinforced, but many bearings in use today use steel shims that are layered with rubber and vulcanized together to form a reinforced rubber block. These types of bearings have a good history and are widely used. The load they can support is generous, as much as 1,000 pounds per square inch and their motion range is a function of the geometric properties of the bearing. Under lateral loading, the bearing will begin to deform. The rubber provides flexibility while the steel shims help control the deformations. In general, the deformation capacity of an elastomeric bearing is roughly half its overall depth.
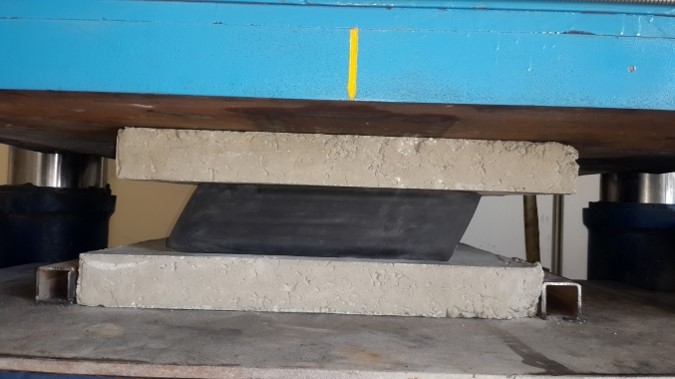
Elastomeric bearings are a good choice for prestressed concrete girders where overall load is not extreme and the motion range is not great. They are also a good choice for splice girders. In longer spans, they can be used to provide a certain degree of seismic isolation – known as poor man’s seismic isolation bearings. The bearing’s flexibility grows proportionally with height, therefore very thick bearings may be used on longer span splice girder bridges to allow for thermal expansion and other movements. The manufacturing method of such bearings essentially makes the sky the limit as far as thickness.
On stiffer and heavier spans, elastomeric bearings are often at their limit. These could be post-tensioned box girder bridges. In these cases, fabric pad bearings are often specified. Fabric pads used a woven cotton duct material that is less flexible than elastomeric bearings, but has much more load carrying capacity. To make up for the loss of flexibility, fabric pad bearings that need to accommodate movement will use sliding surface. Polished stainless steel is applied to one plate, often the sole plate, and the other is coated in PTFE or Teflon – the same stuff that coats your non-stick pans. This low friction surface allows the bridge to move laterally on top of the bearing.
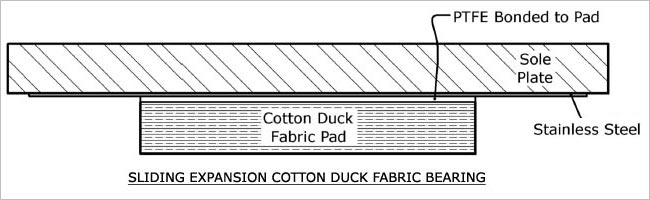
Where the load demands outstrip even the fabric pad or where large amounts of rotational movement must be accommodated, high load multi-rotational (HLMR) bearings are specified. There are several types of HLMR bearings, however most have been superseded by the disk bearing. The disk bearing uses a disk made from urethane to both support loads and allow for large rotational movements. To accommodate lateral movement, a Teflon sliding surface is used. These bearings are common on steel plate girder bridges where the steel’s flexibility often requires high amounts of rotational movement.
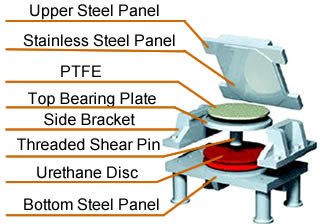
Disk bearings are available in a few different configurations. A pinned type has a large steel pin in the center of the disk which allows for rotations over 360 degrees, but will not allow lateral movement. These are used at a fixed pier which is designed to be hinged but still transmits shear forces to the substructure. A unidirectional type allows for the same 360 degrees of rotation but constrains the lateral movement to one direction via a sliding surface and guide bars. And the third type is the floating bearing, which allows for rotation and movement in more than one direction.
In high seismic regions where the ground motions continue to climb as we learn more about seismicity, engineers must find ways to design and build economic structures that are safe for the traveling public. One way this has been done is by use of seismic isolation. The premise here is that while seismic loading is merely a function of acceleration and mass, neither can really be reduced. The bridge weighs what it does and the seismic acceleration is what the earthquake will throw at it. Or is it?
Seismic acceleration is a further function of structure period. Take a pendulum clock. The period of the pendulum is the time it takes to move form a starting point through a complete range of motion back to that starting point. To speed up the escapement, you move the weight up to shorten the throw. To slow it down, you move the weight down. A shorter throw represents a stiffer system and the period goes down – it ticks faster, thus the time required to make that complete motion goes down. A longer throw represents a less stiff system and the period goes up.
In seismic isolation, the idea is to loosen up the system and push the bridge’s fundamental period out further. As the period goes up, the seismic acceleration goes down. This means there is less lateral load the bridge’s substructure must be designed for and this can in turn reduce the cost of the substructure. It is particularly advantageous in poor soil conditions where either large and/or deep foundations would otherwise be required.

There are a few different types of seismic isolation bearings, but all operate on the same principle. Lateral motion causes the superstructure to move. The bearings are designed to be self-centering, in that they can move but once the motion subsides, the bearing will return to its original position. They function by dissipating the earthquake motion and energy into a back and forth motion – much like a pendulum. Since the geometry of such a bearing can be controlled, so too can the period.
Bearings are a key element in bridges and their inspection and maintenance are paramount. Bearings which no longer function as they should can often allow large stresses to build up in bridge members. This can lead to damage to the bridge. One example of this is old rocker bearings on older bridges. These bearings use pins to either allow only rotation or to allow both rotation and lateral movement. Corrosion and successive painting can lead to these bearings becoming seized up.
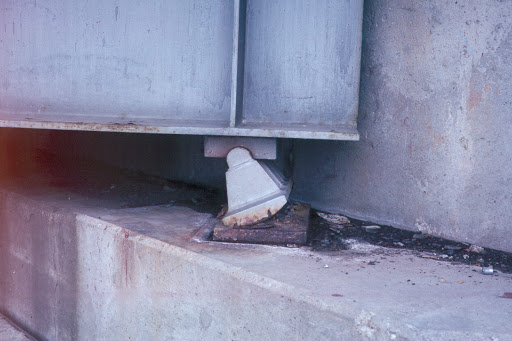
Bridge codes often suggest or even require that designers provide provisions for bearing replacement in the future. Elastomeric bearings have a good track record and do not readily fail if designed correctly, although some pads have been known to ‘walk’ out from under the member. Construction defects can lead to permanent deformation damage to bearings. This can happen where sole or masonry plates are not set true. Disk bearings as well as seismic isolation bearings can be more maintenance intensive than other types of bearings, and while they offer many more benefits over the pin and rocker bearings of the past, they still have a finite service life that may be less than that of the bridge itself.
Like the bearings in your car’s wheels or engine, a bridge’s bearings are critical to its performance. They are a small yet significant part of any bridge and their design must be given proper attention. In bridge models, bearings often represent the structure’s boundary conditions and are often represented by springs or a 1 or a 0. The assumptions made during modeling must be accurate based on the desired bridge behavior and must also be correctly detailed in the bridge plans. Mistakes regarding either can have dire consequences.
So when you look at bridge on your next drive, try to discern the superstructure from the substructure. Think about how the two parts work together, but don’t forget about the glue – or the grease – that exists between the two.
Views: 1339